J. Anim. Physiol. a. Anim. Nutr. 88 (2004), 46–58
2004 Blackwell Verlag, Berlin
ISSN 0931–2439
Receipt of Ms.: 28. 11. 2002
Accepted: 21. 08. 2003
Departments of 1Animal Science and 2Molecular Biosciences, University of California at Davis, Davis, CA, USA
Influences of stage of lactation, teat position and sequential milk sampling on the composition of domestic cat milk (Felis catus)
By K. L. Jacobsen1, E. J. DePeters1, Q. R. Rogers2 and S. J. Taylor1
Summary
Milk from 11 domestic shorthair cats (Felis catus; n ¼ 7 fed dry low-fat diet, n ¼ 4 fed dry high-fat diet) was collected weekly for 6 weeks following parturition, and analysed for total solids (TS), crude protein (CP), fat, lactose and ash. Samples were collected in 1-ml sequential fractions to determine whether within-sampling changes in composition existed. Samples of extracted milk fat were also analysed for fatty acid content. Two commercial kitten milk replacers were analysed according to the same procedures utilized for milk samples. In statistical analyses individual cat, diet, stage of lactation, litter size, and teat position influenced concentrations of milk components; parity and sequential sampling had no effect. Averaged cat milk was 27.9% TS, and 8.7% CP, 12.7% fat, 4.2% lactose and 1.3% ash (on a wet basis). Milk protein percentage increased over lactation for both diet groups, but fat percentage increased only for queens fed the high-fat diet. Milk replacers were lower in fat and protein content than milk from queens, and had considerably lower levels of arachidonic acid. Data from this study contribute to the limited information available regarding the composition of domestic cat milk, and give possible reasons for poor growth occasionally observed in kittens fed unsupplemented commercial milk replacers.
Introduction
Published data on cat milk composition are not only limited, but vary considerably. Many previous researchers reported collecting small milk sample volumes. Adkins et al. (1997) typically collected milk volumes of <1 ml from each cat. Furthermore, older studies (Powers, 1933) failed to provide the number of source queens, and therefore the number of cats contributing to the sample size for milk collection is unknown. Variation in milk composition data across studies is likely due to large potential cat-to-cat variation, nutritional status of queens, composition of the queen’s diet, and differences in analytical procedures for milk analysis. The limited data necessitate additional studies with larger milk sample volumes and number of cats milked.
The influence of teat order on milk composition is unknown. Teat order was proposed to exist merely to minimize competition between kittens before nutritive suckling (Ewer, 1959; DePassille´ et al., 1988). However, kitten survival and growth have not been measured in relation to teat order. There is also no information on potential differences in milk composition associated with individual teats.
Previous research evaluating milk composition of queens was not carried out in relation to the changes in milk composition during a single bout. Changes in fat percentage within a single milking have been evaluated in numerous species, such as the caprine (Calderon et al., 1984), bovine (Whittlestone, 1953), porcine (Whittlestone, 1953; Perrin, 1954), and human (Forsum and Lo¨nnerdal, 1979). Fat content did not vary in sequential milk
U. S. Copyright Clearance Center Code Statement: 0931–2439/2004/8801–0046$15.00/0 www.blackwell-synergy.com
samples in the sow, in contrast to the cow, doe and human, where fat content gradually increased with the progress of milking. There is a paucity of data on the composition of domestic cat milk.
The objective of this study was to determine the composition of queen’s milk over sequential sampling. Animal factors studied included diet, parity and teat location, pectoral teatscompared with inguinalteats. Asecondary objectivewas to comparethecompositionof two commercially available liquid kitten milk replacers with the composition of queens milk.
Materials and methods
Experimental animals
Eleven lactating-specific pathogen-free (no vaccines) domestic shorthair queens (Felis catus), ranging from 1 to 4 years of age, were studied (Table 1). Each queen was fed either low-fat (commercial LF dry expanded diet; Whiskas Original Crave Recipe (Kal Kan Foods Inc., Vernon, CA, USA), n ¼ 7) or high-fat (commercial HF dry expanded diet; Iams (The Iams Company, Dayton, OH, USA), n ¼ 4). Poultry fat was the primary source of fat in both dry diets. Diet chemical composition (%) based on the analyses from the manufacturers for LF were 30.7, 9.4, 6.95, 7.9 and 1.87 and for HF was 33.0, 22.3, 5.90, 7.0 and 1.63 for crude protein (CP), fat, ash, moisture, and crude fibre, respectively. Fatty acid composition of the diets is presented in Table 8. Ingredients for the LF were: chicken and poultry by-products, rice, corn, poultry fat, beet pulp, poultry digest, fish meal, minerals, vitamins and dlmethionine. Ingredients for HF were: chicken, chicken by-product meal, rice, ground corn, poultry fat (preserved with butylated hydroxytoluene), dried beet pulp (sugar removed), fish meal, minerals, dl-methionine and vitamins. A queen’s diet was not changed from that fed during gestation. These animals were a subset of a larger study, and hence the current study is not balanced with number of queens per diet. Queens were milked opportunistically. Litter size varied within each diet group, although it was not a strict criterion for queen selection. Kittens suckled ad libitum during the course of the study, and their body weights were monitored weekly to ensure normal growth.
Milking procedures and sample handling
Milking a queen is typically difficult, and the total volume collected is often small. The following techniques were utilized to allow the collection of large sample volumes.
Table 1. Queen and litter information
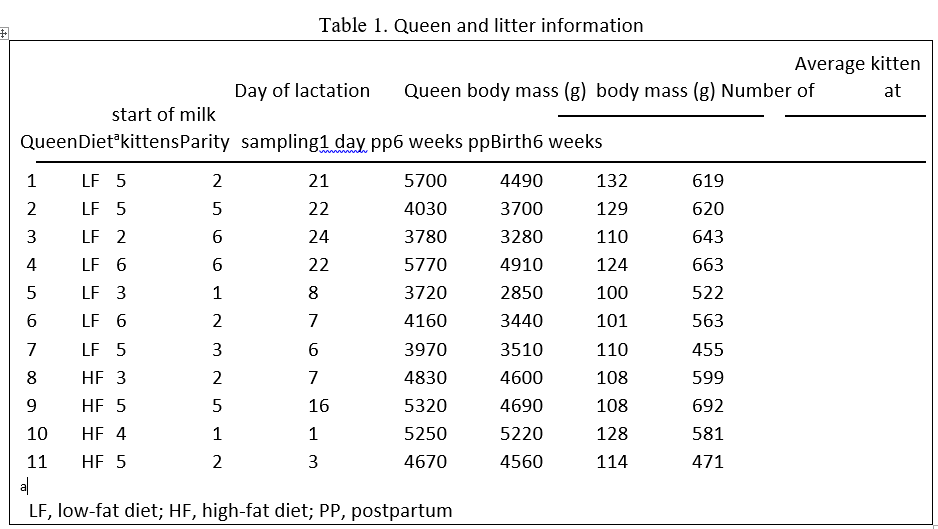
48 K. L. Jacobsen et al.
A queen was separated from her kittens for a period that mimicked the natural nursing interval observed for her kittens’ age to ensure that her glands were full at the time of collection, and the milk collected was not merely residual. These separation periods were 30 min for litters 2-weeks-old and younger, and 45–60 min for older litters. Frequent massaging of the mammary glands during milking helped to sustain ejection without administering additional oxytocin. A queen objecting to the sitting position often would quickly settle down, ‘recline’ with all legs stretched out, and knead once her glands were massaged, simulating the natural kneading by the kittens. The process of milking a single queen required approximately 45–60 min, volumes collected amounted to 12–15 ml. However, between 20 and 25 ml were collected from several queens.
Each queen was subcutaneously injected at the scruff of the neck with 5 units of oxytocin (0.25 cc of a solution containing 20 units of oxytocin per ml) using a 2.54 cm, 25-gauge needle was used for all injections. Milk collection started 10 min following the oxytocin injection. Milk was collected by manual expression into preweighed 5-ml plastic tubes. Volumes of 1 ml were collected at a time, with the front teats being collected separately from the back teats. All functional teats were milked, with an attempt to maintain a constant amount from each teat for a given 1-ml volume. Following collection each tube was weighed. After collection, the tubes were placed in an insulated container for transport to the laboratory, where subsampling occurred either immediately or the next day. If the milk was not subsampled until the next day, a bacteria-inhibiting preservative pellet (Broad Spectrum Microtabs II, D&F Control Systems, Inc., San Ramon, CA, USA) was added to each tube before the tubes were placed in a refrigerator.
The milk, either fresh or cooled from the previous day, was first warmed in a 40 C water bath and mixed gently before subsampling. For lactose determination, 50 ll of milk were diluted with double-deionized water to a volume of 2.5-ml. Aliquots of 0.5 ml were then pipetted for fat and nitrogen (N) fractions, which were kept frozen until analysis. Since it was suspected that within-sampling changes in composition would most likely occur with fat content, collected volumes smaller than 1 ml were subsampled with priority given to fat determination. Any remaining milk in each tube was then pooled for dry matter (DM) and ash determinations. Each sample for each queen and each week of collection were kept separate for all such poolings.
Analytical procedures
Frozen milk samples were rapidly thawed in a 40–45 C water bath just prior to the time of analysis. For N determination, the samples (262 total) were digested in 5 ml of a 60 : 40 sulphuric acid : hydrogen peroxide solution (v : v) with approximately 1.60 g of Kjeldahl catalyst mixture (97% sodium sulphate and 3% copper sulphate) and boiling chips in 75-ml block digester tubes. Following digestion and cooling the tubes were brought up to volume with double deionized water. After mixing, portions of the solutions were then transferred to 5-ml plastic tubes and later analysed for N content using an AutoAnalyzer (Technicon Corporation, Tarrytown, NY, USA), according to AOAC official method 976.06 for protein in animal feed (aoac, 1985aoac, 1985). Ammonium sulphate, digested in the same manner as the milk samples, was used to construct the standard curve. CP percentage was calculated from total percentage of N (%CP ¼ %N · 6.38).
Total lipids were measured using a hexane/ethanol extraction (313 samples total), based on the milk fat extraction procedure as described by Erickson and Dunkley (1964). The extracted fat was transferred to a 50-ml glass screw-cap centrifuge tube. To each tube 0.5 ml of saturated sodium chloride and 9 ml of ethanol for extraction were added. The ethanol for extraction was prepared by combining 1800 ml of 95% ethanol with 120 ml of 1 m hydrochloric acid. Each tube was then stoppered with a Teflon-lined cap and vortexed for 15 s. Hexane was added (5 ml) with a volumetric pipette. The tubes were capped and placed on a shaker for 10 min, followed by centrifugation at approximately 300 G for 10 min. From each hexane layer, a 1-ml sample was obtained using a volumetric pipette, and the sample was transferred to a pre-weighed aluminium pan. Following evaporation of the hexane the pans were placed in a 100 C forced-air drying oven for 15 min, and then placed in a desiccator for 15–30 min. Pans were weighed and the percentage fat calculated. The entire hexane layers remaining for selected samples (75 total) were transferred to small glass screw-cap tubes with Teflon-lined stoppers. These tubes were then placed in a freezer ()80 C) until gas–liquid chromatography (GC) analysis was performed. A 100 m capillary column (SP-2560, 100 m · 0.25 mm; Supelco, Inc., Bellefonte, PA, USA) was used for GC analysis of fatty acids (Crocker et al., 1998).
Lactose content (272 samples total) was determined using an AutoAnalyzer, which measured the reducing sugar content (Conneta et al., 1970). Samples for total solids (TS) (49 total) were placed in pre-weighed aluminium pans, dried in a 100 C oven for 4 h, and allowed to cool in a desiccator before weighing. The dried milk samples were then placed in a muffle furnace at 550 C for 12 h to determine total ash content.
Two commercially available liquid kitten milk replacers were used for comparisons with cat milk. Two samples of each milk replacer were purchased at different times and analysed. Each replacer was analysed for TS, fat, N, lactose and ash using procedures described for milk. Additionally, the extracted fat from the milk replacers was subjected to fatty acid analysis.
Data analysis
Data were analysed by anova with Bonferroni post hoc tests using the spss statistics program (version 10.0 for Windows, Chicago, IL, USA). Significance was set at p £ 0.05 while a trend was p > 0.05 and £0.10. Cat, diet, stage of lactation, teat position (front vs. back), parity, and sequential fraction collected were used as independent variables in each analysis. Although queens have the potential to behave differently, equal numbers of sequential fractions were not collected each week from each queen. Thus, in order to avoid over-representing the ‘well-behaved queens’ (i.e. those that permitted the most sequential fractions to be collected) in the data set, thereby skewing data to favour those queens, data from individual fractions were averaged for each queen at each week postpartum, keeping teat position separate. All data from sequential fractions were kept separate for each queen at each week postpartum only for statistical analyses using sequential fraction as the independent variable. However, for statistical analyses with individual cat, diet, weeks postpartum, parity, litter size, and teat position as independent variables, a data set based upon calculated averages was utilized, so that each queen contributed one average front teat set of values and one average back teat set of values at each week postpartum.
Results
General composition
Body weight gain of the kittens was not affected by the diet fed to their dams (Table 1). Weight loss of queens during the first 6 weeks of lactation was less for cats fed HF compared with LF. The queens fed HF probably relied less body reserves than LF queens. On average, during the 6-week lactation period the LF queens lost 660 g of body weight compared with 252 g for HF queens.
Results from anova are shown in Table 2. CP percentage started to increase around 3 weeks postpartum, and continued to steadily increase until the end of lactation (Table 3). Fat percentage decreased slightly by week 3 of lactation, and gradually increased until by week 6 when it was slightly higher than the initial level. TS changed with increases and (or) decreases in fat or protein percentages. Lactose levels increased until week 4 followed by a
Table 2. Significance (p-values) of variables to concentrations of crude protein, fat, lactose and ash in queen milk, as measured by ANOVA
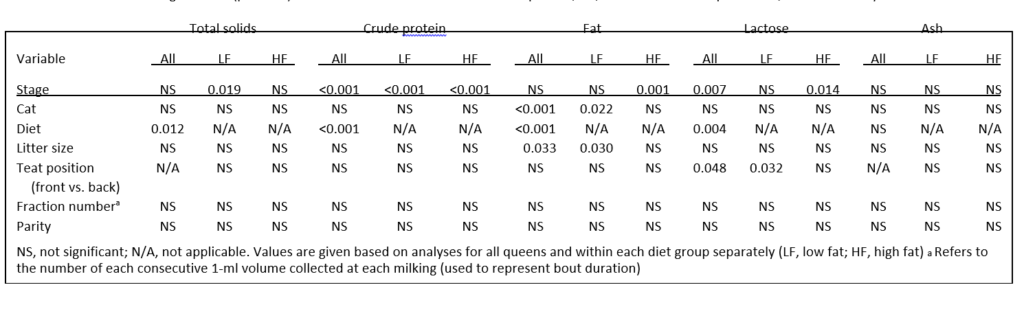
Table 3. Gross composition of all queen milk (mean ± SEM) throughout lactation
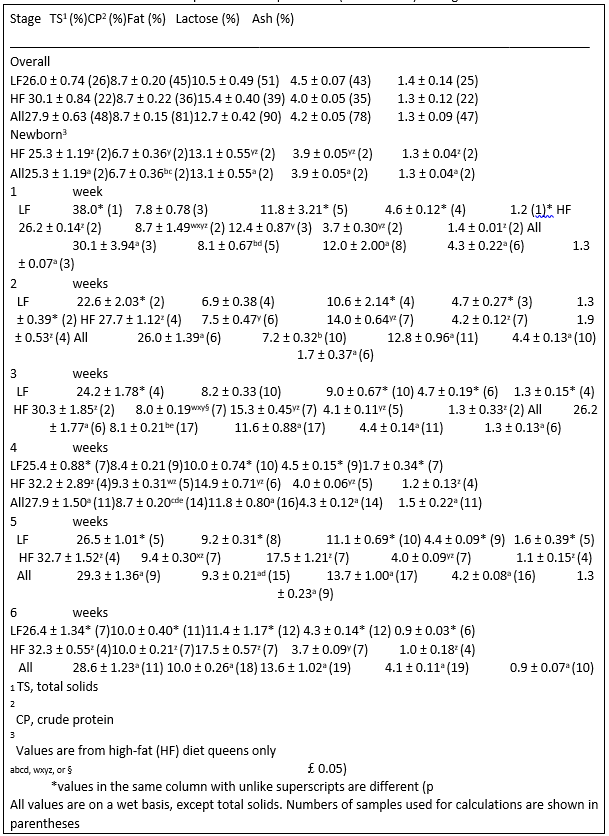
decrease throughout the remainder of lactation. Among all queens, ash concentrations in milk remained fairly constant throughout most of lactation.
The TS content of milk from HF queens was greater than from LF queens when samples from queens fed each diet was considered separately. This difference was mainly the result of increases in milk fat in HF queens (p < 0.001); LF and HF queens had similar milk protein content, but LF queens had higher levels of lactose (p ¼ 0.004) in their milk than HF queens. Lactose percentage peaked at week 2 of lactation followed by a gradual decreases throughout the rest of lactation, although stage was significant only in HF queens (p ¼ 0.014). A similar trend was also seen for ash levels, although stage was not significant in either diet group. Protein increased in milk from both LF and HF queens (p < 0.001, either diet group) with advancing stage of lactation. Fat composition changes differed although, with an increase in percentage during lactation seen only in HF queens (p ¼ 0.001).
Litter size affected milk fat percentage (p ¼ 0.033). Gross milk composition for all queens and for those fed each separate diet were compared (Table 4). Litter size had no
Table 4. Gross composition of queen milk (mean ± SEM) based on litter size
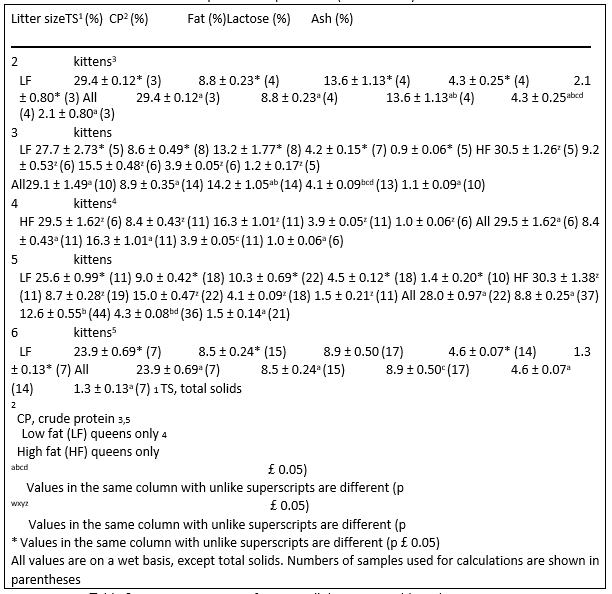
Table 5. Gross composition of queen milk (mean ± SEM) based on teat position
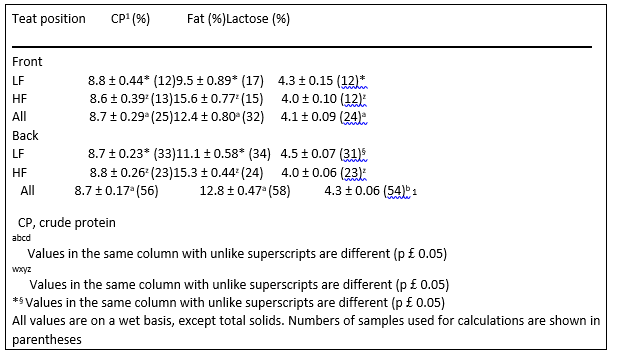
effect on TS, CP, lactose, or ash in all queens or within each diet group. However, litter size did affect milk fat percentage in LF litters (p ¼ 0.03). Generally, milk fat percentage decreased as litter size increased, most likely corresponding to a dilution of fat that occurs with a larger litter’s demand for a higher total volume of milk.
Back teats from LF queens had higher lactose concentration than front teats (p ¼ 0.032, Table 5). Ewer (1959) proposed that back teats were superior to the front teats in terms of milk volume produced. Considering lactose’s role in osmoregulation and hence volume
Table 6. Gross composition of queen milk (mean ± SEM) based on sequential fraction
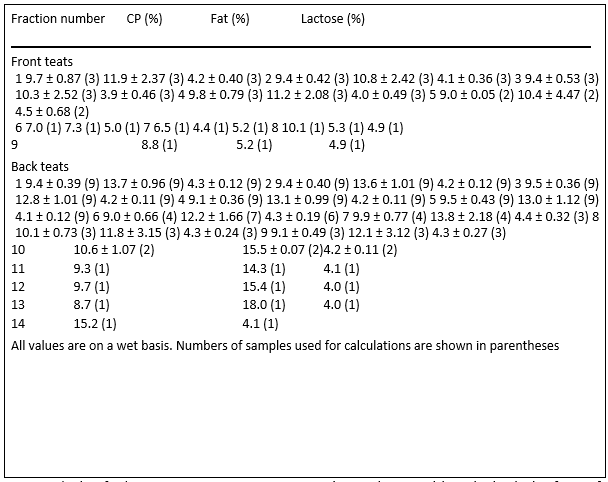
secreted, this finding may seem to support Ewer’s conclusion, although the lack of specific milk volume measurements necessitates additional investigation.
Gross milk composition of milk samples did not change with sequential collection of milk during the collection period (Table 6). Parity also did not affect gross milk composition of queens (Table 7).
Gross compositions of two commercially available liquid kitten milk replacers are shown in Table 8. Relative to queen milk, both milk replacers have similar percentages of lactose and ash. Percentages of protein, and especially fat, of milk replacers were low compared with levels found in milk from queens.
Fatty acid composition
The fatty acid composition of milk fat did not differ for any of the independent variables, and an average profile is therefore reported for milk fat from queen’s milk (Table 8). The fatty acid composition of the LF and HF diets is also presented. There were numerical differences in fatty acid composition between the two milk replacers, most noticeably with C18:1 n9c and C18:2 n6c. Note that the fatty acid profiles for each diet are presented as percentages of the total diet DM, not of the total dietary fat. Primary fatty acids in the fat from queen milk, replacers, and diet DM were C16:0 (palmitic), C18:1 (oleic) and C18:2
(linoleic) acids.
A long capillary column was used for GC analysis to ensure that arachidonic acid (C20:4 n6) was clearly separated. The D6-desaturase activity is low in cats, and therefore desaturation of linoleic acid to arachidonic acid is low in cats (Rivers et al., 1975; Pawlosky and Salem, 1996). Dietary sources of arachidonic acid are generally considered essential.
Table 7. Gross composition of queen milk (mean ± SEM) based on parity
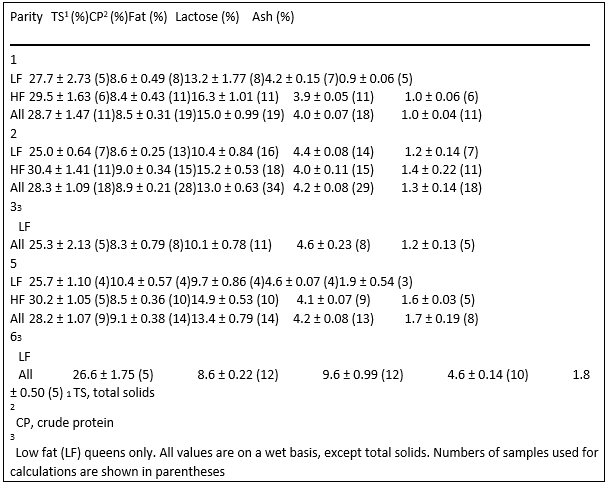
It was of interest to not only determine the fatty acid profile of milk fat from queens, but also to compare the average fatty acid composition to those of the milk replacers, with special attention paid to arachidonic acid content. The average fatty acid composition of milk fat from queens was compared with the fatty acid composition of milk replacers on a numerical rather than a statistical basis. The predominate fatty acids in the milk replacers were C16:0, C18:1 and C18:2, similar to cat milk. However, there were some short chain fatty acids (C4:0–C8:0) found in the KMR replacer that were not present in cat milk fat. Arachidonic acid (C20:4) was present in the milk replacers, but at much lower concentrations than found in the milk fat of queens. While the milk fat contained 0.81% arachidonic acid, the milk replacers’ fat contained only 0.08 or 0.13%. The HF diet had considerably more arachidonic acid as percentage DM than LF, but there was no notable difference in arachidonic acid levels in milk fat from HF queens when compared with milk fat from LF queens.
Discussion
Milk composition was affected by stage of lactation (Table 3). For all queens combined, protein content of milk increased (p < 0.001) while lactose content increased (p < 0.007) to week 3 followed by a decrease as lactation progressed. Milk protein percentage increased significantly with advancing lactation for LF and HF queens, but fat percentage increased only for HF and TS increased only for LF queens. Changes in milk composition with stage of lactation have been observed for cows and rabbits (Touchberry, 1974; Davies et al., 1983; Mepham, 1987). A summary by Oftedal (1984b) described general trends in milk composition over lactation for an extensive list of species. The single reference for the domestic cat reported that milk fat and sugar levels were unchanged, with only increases in protein over lactation.
The lack of a significant effect of sequential fraction collection on milk composition suggests that small volumes of milk can be collected and be representative of what kittens
Table 8. Composition of milk replacers, queen’s milk, and queen diets
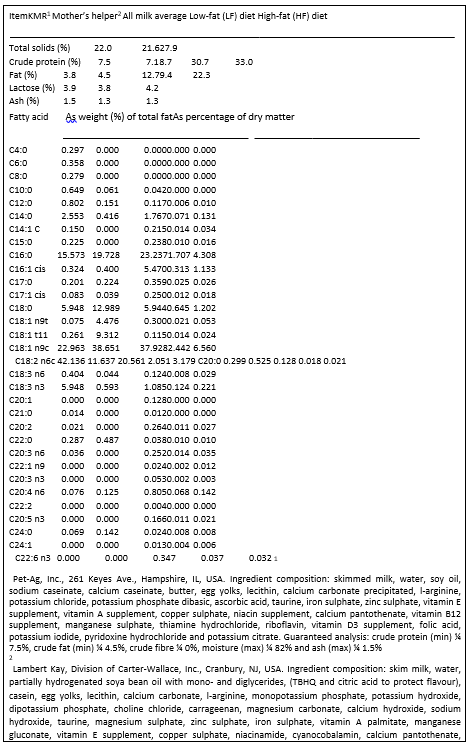
receive during a nursing bout. However, because the collection of milk depends on a continuous letdown, it was not possible to know whether glands had been completely evacuated. Once the queen was returned to her kittens, she almost always immediately nursed them, suggesting that her glands still contained some milk. Further studies involving both milk collection in sequential fractions from single teats combined with the use of weigh-suckle-weigh or isotope methods to estimate a kitten’s milk intake, and hence estimate gland capacity, would be needed to further support the finding that milk composition does not change during the milking process.
There is no consensus on the gross composition of queen’s milk in the literature. Many studies were based on small sample volumes, which may not have been representative of the milk available in the gland. Protein percentage ranged from approximately 5.7 (Keen et al., 1982) to 11% (Schmidt, 1971), with most values at 7–7.5% (Powers, 1933; Ben Shaul, 1962; Baines, 1981; Iben and Leibetseder, 1994; Adkins et al., 1997). Fat percentage ranged from approximately 4 (Baines, 1981) to 11% (Schmidt, 1971; Oftedal, 1984b; Oftedal and Iverson, 1995). While ash levels were most frequently 0.7–1%
(Powers, 1933; Ben Shaul, 1962; Oftedal, 1984b; Iben and Leibetseder, 1994; Oftedal and Iverson, 1995; Dobenecker et al., 1998), levels of 3–4% were also reported (Baines, 1981).
Despite the large variation in percentage of protein, fat and ash, with the exception of Widdowson (1964) who reported concentrations of 10% lactose, most lactose concentrations were between 4 and 5% in all references cited above. Caution must be used when comparing these values, however, as some investigators measured total carbohydrate content rather than just lactose. In the interest of accuracy in reporting sugar content, lactose levels alone are not adequate, as carnivore milk sugar could contain other sugars such as mono- and oligosaccharides (Jenness, 1974; Oftedal, 1984b; Mepham, 1987; Oftedal and Iverson, 1995). However, lactose percentage provides a basis for overall comparison among investigators.
The variation in milk composition across studies could be a result of animal variability, analytical and sampling procedures, stage of lactation and diet. For example, Adkins et al. (1997) reported true protein and not total CP, and they converted percentage N to true protein using a correction factor of 6.25 and not 6.38. The latter typically used for milk N while the former is used for feedstuffs. Milk fat percentage was affected by diet (Table 3), but not all previous studies reported the fat composition of diets. Diet composition fed should be considered in all studies reporting milk composition of queens as well as stage of lactation to allow comparison of results across studies. Procedural differences are likely if the milk fat was analysed spectrophotometrically without utilizing reference standards suitable for cat milk analysis (Oftedal, 1984a,b). Without such standards, dramatically underestimated values are possible with assays developed for cow’s milk.
The fatty acid composition of milk fat from the cat was similar to the same general pattern that pertains to carnivores. Milk fat was high in C16:0 (palmitic), C18:1 (oleic) and C18:2 (linoleic) acids. There is probably little mammary synthesis of the fatty acids, and so composition would closely resemble dietary and depot fat (Glass et al., 1967; Iverson and Oftedal, 1995). Cat milk fat was analysed previously, but most studies used analytical procedures that did not measure the long chain fatty acids. As a result, there are few published estimates of arachidonic acid content of queen’s milk. Dobenecker et al. (1998) analysed cat milk fat and found 20.6% linoleic acid and 1.7% arachidonic acid. The linoleic acid was similar to the level observed in the present study, but arachidonic acid was higher than the 0.81% observed. Since the composition of the milk fat is influenced by diet, it is possible that the diets in Dobenecker et al. (1998) and the current study differed in arachidonic acid content. The rate of arachidonic acid synthesis is low in cats so dietary concentration will impact depot and milk fat content (Pawlosky et al., 1994).
Implications for hand-rearing kittens
Based on gross composition analyses, both commercially available liquid kitten milk replacers considered in the present study had ash and lactose contents within the normal ranges measured in cat milk. Protein and fat percentages of milk replacers were, however, comparatively low. These values resembled those found in early cat milk studies, and therefore it is likely that the replacer formulations were based on these earlier studies.
Comparison of gross analyses for cat milk replacer and fatty acid profiles of cat milk (Table 8) may shed some light on why many orphan kittens die or grow poorly on unsupplemented commercially available milk replacers. Those who have successfully handreared kittens have often used homemade recipes containing whole or condensed milk and egg (Baines, 1981; Remillard et al., 1993). Iben and Leibetseder (1994) compared growth rates of kittens fed either a prepared feline milk replacer or a less concentrated homemade replacer whose composition was similar to that of KMR. Lower growth rates frequently seen with replacer-fed kittens are probably the result of lower caloric density resulting from less fat and protein intakes (ash and estimated carbohydrate concentrations are similar to those in cat milk). Although present, arachidonic acid content was much lower in either milk replacer compared with levels found in queens’ milk in the present study, which could additionally contribute to compromised growth rates and health.
Conclusions
Composition of milk from queens was affected by diet, stage of lactation, litter size and teat position. Sequential sampling of milk during the milking process did not affect composition. These results support the validity of previous research involving smaller milk sample sizes and suggest that differences in milk composition observed for various studies may be a result of diet and methods of milk analysis.
Acknowledgements
The authors thank the staff at the U.C. Davis Nutrition and Pet Care Center, notably Debbie Bee and Jennifer Bones, as well as milking assistants Daphne Livoni and Grace Ragasa. Financial support was provided by the California Agricultural Experiment Station as well as partial funding provided by the George and Phyllis Miller Feline Health Fund of the San Francisco Foundation and the Center for Companion Animal Health, School of Veterinary Medicine, University of California, Davis.
References
Association of Official Analytical Chemists (AOAC), 1985: Official Method 976.06, Protein in Animal Feed (Semiautomated Method), 16th edn. AOAC International, Arlington, VA, USA
Adkins, Y.; Zicker, S. C.; Lepine, A.; Lo¨ nnerdal, B., 1997: Am. J. Vet. Res. 58, 370–375. Baines, F. M., 1981: J. Small Anim. Pract. 22, 555–578.
Ben Shaul, D. M., 1962: Int. Zoo Yrbk. 4, 333–342.
Calderon, I.; DePeters, E. J.; Smith, N. E.; Franke, A. A., 1984: J. Dairy Sci. 67, 1905–1911.
Conneta, A., Stookey, L., Zehnder, H., 1970: An automated system for the determination of milkfat, protein, and lactose in milk. In: Adelman, M., Barton, E. C., Buggie, F. D., Conetta,
A., Erdich, M. M., Gasko, R. M., Golin, J. E., Greenfield, R., LaPerch, F., Perlman, L., Salpeter, J., Smythe, W., Stark, E. W., Zehnder, H. (eds) Advances in Automated Analysis, Vol 2. Technicon International Congress. 1970, Industrial Analysis, Futura Publishing Company, Mount Kisco, NY, pp. 81–85.
Crocker, L. M.; DePeters, E. J.; Fadel, J. G.; Perez-Monti, H.; Taylor, S. J.; Wyckoff, J.; Zinn, R. A., 1998: J. Dairy Sci. 81, 2398–2407.
Davies, D. T., Holt, C., Christie, W. W., 1983: The composition of milk. In: Mepham, T. B. (ed.), Biochemistry of Lactation, Elsevier, Amsterdam, pp. 71–117.
DePassille´, A. M. B.; Rushen, J.; Hartsock, T. G., 1988: Can. J. Anim. Sci. 68, 325–338.
Dobenecker, B.; Zottman, B.; Kienzle, E.; Zentek, J., 1998: J. Nutr. 128, 2618S–2619S.
Erickson, D. R.; Dunkley, W. L., 1964: Anal. Chem. 36, 1055–1058. Ewer, R. F., 1959: Behaviour 15, 146–162.
Forsum, E.; Lo¨ nnerdal, B., 1979: Nutr. Rep. Int. 19, 815–819.
Glass, R. L.; Troolin, H. A.; Jenness, R., 1967: Comp. Biochem. Physiol. 22B, 415–425.
Iben, C.; Leibetseder, J., 1994: J. Nutr. 124, 2630S–2632S.
Iverson, S. J., Oftedal, O. T., 1995: Comparative analysis of nonhuman milks: B. Phylogenetic and ecological variation in the fatty acid composition of milks. In: Jensen, R. G. (ed.), Handbook of Milk Composition, Academic Press, New York, pp. 789–827.
Jenness, R., 1974: The composition of milk. In: Larson, B. L., Smith, V. R. (eds), Lactation: A Comprehensive Treatise, Vol 3. Academic Press, New York, pp. 3–96.
Keen, C. L.; Lo¨ nnerdal, B.; Clegg, M. S.; Hurley, L. S.; Morris, J. G.; Rogers, Q. R.; Rucker, R. R., 1982: J. Nutr. 112, 1763–1769.
Mepham, T. B., 1987: The composition of milks. In: Physiology of Lactation, Open University Press, Philadephia, pp. 30–50.
Oftedal, O. T., 1984a: J. Nutr. 114, 803–812.
Oftedal, O. T., 1984b: Symp. Zool. Soc. Lond. 51, 33–85.
Oftedal, O. T.; Iverson, S. J., 1995: Comparative analysis of nonhuman milks: A. Phylogenetic variation in the gross composition of milks. In: Jensen, R. G. (ed.), Handbook of Milk Composition, Academic Press, New York, pp. 749–789.
Pawlosky, R. J.; Salem, N. Jr, 1996: J. Nutr. 126, (Suppl. 4), 1081S–1085S.
Pawlosky, R.; Barnes, A.; Salem, N. Jr, 1994: J. Lipid Res. 35, 2032–2040.
Perrin, D. R., 1954: J. Dairy Res. 21, 55–62. Powers, G. F., 1933: J. Ped. 3, 201–216.
Remillard, R. L.; Pickett, J. P.; Thatcher, C. D.; Davenport, D. J., 1993: Am. J. Vet. Res. 54, 901– 907.
Rivers, J. P. W.; Hassam, A. G.; Alderson, C., 1975: Proc. Nutr. Soc. 35, 67–68A.
Schmidt, G. H., 1971: Biology of Lactation. W.H. Freeman Company, San Francisco, p. 2.
Touchberry, R. W., 1974: Environmental and genetic factors in the development and maintenance of lactation. In: Larson, B. L., Smith, V. R. (eds), Lactation: A Comprehensive Treatise, Vol 3. Academic Press, New York, pp. 349–381.
Whittlestone, W. G., 1953: J. Dairy Res. 20, 146–153.
Widdowson, E. M., 1964: Food, growth and development in the suckling period. In: Graham-Jones, O. (ed.), Canine and Feline Nutritional Requirements, Pergamon Press, Oxford, pp. 9–17.
Author’s address: Edward J. DePeters, Department of Animal Science, One Shields Ave., University
of California at Davis, Davis, CA, USA 95616-8521. Tel: 530-732-1263; Fax: 530752-0175; E-mail: ejdepeters@ucdavis.edu